Seeking for a fifth force at the CERN Large Hadron Collider
Elementary particles are known to interact via three fundamental interactions, namely the strong force, the weak force and electromagnetism. The fourth fundamental interaction, gravity, is left apart in the miscroscopic world, as totally negligible.
However, physicists like to consider options for new interactions. And since ‘more than four’ starts with ‘five’, I will discuss in this post how a fifth force is searched for at the Large Hadron Collider, the LHC, at CERN.
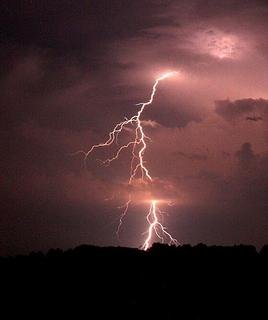
[image credits: Wikimedia]
Why do we have only four fundamental interactions? We actually do not know, and there could actually be more than four of them. The extra ones would be just hiding.
Physicists therefore try to find some proofs (i.e. facts) about the existence of a new fundamental interaction. For the moment, all searches however indicate that there is not a single sign of such a new force.
The latter is therefore more and more constrained experimentally, but the hunt is not over!
I will explain below how new forces are searched for at the LHC.
But before, I will go back to some basic stuff. If you feel comfortable with that, please go directly to the last section of this post.
ELEMENTARY PARTICLES - A SHORT DEFINITION
Before starting to discuss the world of the elementary particles, it is good to define what it is.
The fundamental world is comprised of three types of particles. We have the particles that belong to what is generically called the matter sector, the particles connected to the mediation of the fundamental interactions and the Higgs boson.
I will restrain myself from entering into any nasty detail, as this is not the topic of this post. I however recommend this older Steemit article of mine for more information. A summary is provided below.
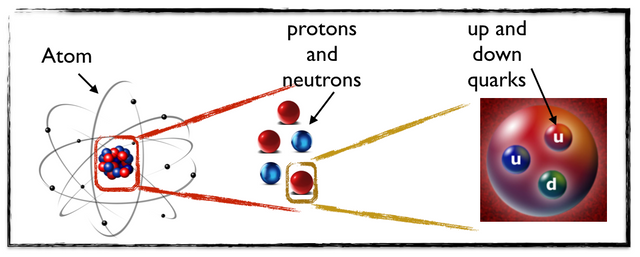
[image credits: homemade (from stuff available everywhere)]
The particles of the matter sector consist of the particles that are the basic building blocks of matter. We focus of course on the smallest bits of matter, that are the up and down quarks and the electrons.
Up and down quarks (rightmost part of the above picture) are the constituents of the protons and neutrons (middle part of the above picture), and neutrons and protons make what is known as atomic nuclei (for example, the core of the atom on the leftmost part of the above picture). Finally, atoms (leftmost part of the above picture) are made of their nucleus, as well as some orbiting electrons.
On top of this, we need to include neutrinos that play a big role in the weak interactions.
The first generation of fundamental objects is made of the most basic objects introduced above: the up and down quarks, the electron and the associated neutrino.
Nature however likes to have fun of us and decided to multiply all of this by three. Three copies are better than one.
If you know why, this is for you! I personally do not know why.
THE THREE FUNDAMENTAL INTERACTIONS (THAT WE CARE ABOUT)
So we have 12 basic particles (3 copies of 4 guys), as said above, that interact via three of the fundamental interactions, as also said above.
The way to model those interactions is to use the concept of gauge symmetries. I will once again ignore all details and provide a summary below.
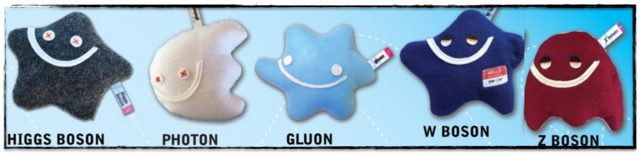
[image credits: Particle Zoo]
What is important to know is that two particles are said to interact when they exchange a gauge boson. The gauge bosons are by definition the particles responsible for the implementation of the interactions in the quantum world.
Having one interaction means that one or more gauge bosons have been exchanged.
We have a bunch of these gauge bosons in the Standard Model, namely the photon (electromagnetism), the W-boson and Z-boson (weak interactions) and the gluons (strong interactions). For instance, an electron and a positron are said to interact electromagnetically if they exchange a photon.
In the case of a fifth force, an extra boson is hence needed.
I will omit from the discussion anything about the Higgs boson. For more information, I refer to here.
SEARCHING FOR EXTRA INTERACTIONS
After this very long introduction, you now know how extra interactions work in particle physics: they work in the same way as the three other interactions. Particles will interact by exchanging a new boson that is associated with the extra interactions.
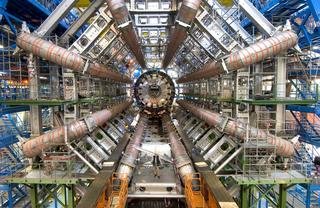
[image credits: ATLAS@CERN]
Therefore, in order to look for a new force, it is sufficient to look for the corresponding boson. There are thus numerous searches for extra bosons at the LHC, at CERN.
These searches rely on the fact that quarks and leptons (i.e., electrons, muons, taus and neutrinos) interact via the new force. This assumption leads to two important points.
First, we can produce the new boson at the LHC, as the colliding protons are made of quarks. Second, a produced new boson can decay into quarks or leptons.
It is thus sufficient to look for the final-state products to reconstruct the presence of an intermediate extra boson.
DISCUSSION: A PRACTICAL EXAMPLE
More precisely, let us pick up an example: a pair of muon-antimuon.
In the Standard Model, a muon-antimuon pair can be produced via weak and/or electromagnetic interactions. One of the quarks from one of the colliding protons collides with one of the antiquarks from the other proton. This gives either a photon (electromagnetism), or a Z-boson (weak interactions), that then decays into a muon-antimuon pair.
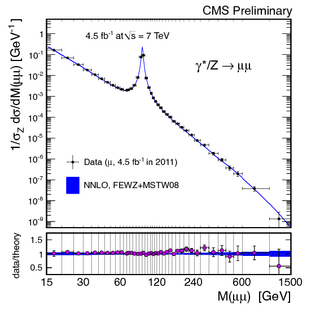
[image credits: Inspire]
We can measure the mass of the system made of the muon and the antimuon. In the boring case where nothing new exists, the probability to get a large mass is smaller and smaller with the mass value.
This is illustrated on the figure on the right where the mass of the muon-antimuon pair lies on the x-axis, and the rate to produce a pair of particles with a given mass is shown on the y-axis.
But then, what is this bump in the middle that seems to ruin my explanation? Such a bump is connected to the existence of the Z-boson: it lies exactly at the mass of the Z-boson on the x-axis.
Anywhere else, the intermediate photon or Z-boson is actually virtual. This can be seen as disturbance in the quantum fields that are the real stuff behind all of this. In other words, the intermediate state does not exist but quantum field theory makes the process is possible.
Now, in the case where we would have a fifth force, we just should see another bump.
Here we are! Now you know how extra forces are searched for at the LHC! It is done with electron-positron pairs, muon-antimuon pairs, tau-antitau pairs, jet (objects originating from a quark-antiquark system) pairs, etc.
The absence of any bump for all existing searches means that either there is no fifth force at all, or the boson is heavier so that the LHC cannot probe it. Or, the boson must be stealthy. This will be the topic of another post (this one is already long enough) :)
For more discussion on this topic (or anything related to science), please join us on steemSTEM. SteemSTEM is a community driven project which seeks to promote well written/informative Science Technology Engineering and Mathematics postings on Steemit. More information can be found on the @steemstem blog.
We are currently consolidating our curation trail by different means. For more information, please contact us on the chat.
@justtryme90: How good am I now, in hiding (several) crazy stuff? Can you catch them all? :p
Hello,
For more information.
The stealthy boson!!
Man, as if it's not hidden enough already!?
Courtesy of @joshoeah
There are actually way to make it hidden in the sense that it is in principle reachable at the LHC in terms of its mass, but the way it couples to the other particles make it not so easily detectable. For instance, if the boson is leptophobic, it does not couple to electrons and the golden channel to find it... is just useless.
Great post as always. Would be an interesting change to the physics books if we find a fifth fundamental force
This will be an interested change on the one hand, but one of the possible change we are expecting. There are actually books on beyond the Standard Model physics that include that option :)
In your post you mention the peculiarities of the number 3 in the standard model. I've thought about this before, and probably/possibly totally unrelated, but I've thought of past/present/future when this has been brought up. I'm sure I'm not the only one, and maybe because it is just something else that "occurs in three parts," but maybe there is some connection?
I am not a big fan of numerology. There are several numbers that appear as such in the Standard Model and for which we are still looking for an explanation :)
I love these posts, short, straight to the point, and when I have finished reading them I know something new: often the mass/probability diagram are shown and it is said that when there is a bump, it is evidence of the existence of a particle, while never explaining what was actually measured (description of the axis). You managed to do that in a just few sentences: chapeau!
A question though, within the hypothesis that dark matter is made of heavy particles.
If these particles are heavy, it is because they are stable, they cannot decay into lighter ones (into particles of standard matter for example).
The reason for that would be the existence of a “parity charge”, a quantity that must be conserved, like for example the lepton number or the electric charge with standard matter.
If that is the case, how could a quark-quark collision produce a dark matter boson? In such an event, the parity would not be conserved… So within this hypothesis, quark-quark collisions would never be able to prove the existence of a "dark force"… if a new boson is discovered one day with this method, it wouldn't be related to dark matter.
Is my reasoning correct, or have I missed something?
I am not fully satisfied with my description and I still want to improve it. I liked it when I posted my article, but today, two days later... I think I can do better. Maybe in my next post on extra bosons, later this week or next week.
Dark matter can actually be light as well. The range allowed for its mass spans many many orders of magnitude (please check here for instance).
In many models, this is the case. The decay process is just forbidden by some symmetry and therefore does not happen. (By the way, the lepton number is accidentally conserved in the Standard Model; there are high energy processes where it is violated.)
The point is that a quark-antiquark collision produces a pair of dark matter particles, so that the parity is conserved. Only the production of a single dark guy is forbidden.
What this new force of interaction may be? Are we going to discover a new kind of quantum force? Can this be related to dark matter/energy?
Btw great article. It opened up my mind and cooked more curiosity and questions as well. :p
And sorry for my noobish questions. 😅
It could be related to dark matter. For instance, the new force may be a dark force (if you remember my older posts on dark photons). What we know on dark matter is that it does not interact electromagnetically. Except this, anything is possible, including a dark matter particle interacting via a new force. But in this last case, the boson mediating the force may be part of the stealthy ones :)
In your post, you said that that quarks and leptons (i.e., electrons, muons, taus and neutrinos) interact via the new force.
I thought that neutrinos doesn't interact at all with other particles.
Can you explain how this is possible or a link to a post that you explained this process.
Many thanks!
Great post, enjoyed reading it XD
Neutrinos interact via week interactions. Please have a look here. In the case of a new force, neutrino can be sensitive to it or not. This depends on the definition of the new force.
Do not hesitate to ask for more information if you need :)
Even nutrinos have different flavours. Did not know about that.. Guess youtube science videos don't provide all the details :(
This probably depends on the video, I guess. I have never looked for youtube videos (I don't like videos in general), but I am pretty convinced good ones can be found. The only problem being that without a particle physics background, it may be complicated to tell which one is good and which one is not so good.
I had a physics background till end of college and the subject that I was most passionate about was quantum physics which was the option I took.
It was a difficult subject but it really got me thinking about the nature of the world.
Being busy, I prefer to the videos to keep in touch in the advances in physics. I like to know what new stuffs physics is helping to discover and help me think outside the box. I cook and sleep watching videos on science.
I guess I will need to do some more research after getting new knowledge but time is not always on my side my friend.
That's why your posts come in beneficial for me XD
Thank you very much! :)
Thanks for the post :) Also I love those Particle Zoo plushies! We have the magnet set version - our fridge looks like the LHC exploded all over it ;)
I am still hesitating to buy the plushes. They are cool, for sure, but not so cheap. (I also have the magnets on my fridge :p )
Yeah they are pricey, especially with the shipping to the UK! Can’t quite justify it yet :P
I think I’m beginning to pick up the fragments of my broken physics life. Before, I wouldn’t sit to read anything in particle physics. But here I am, reading particle physics and understanding a bit. All thanks to you @lemouth
You are welcome :)
Heavy stuff, I like it :-)
Thank you! So heavy that the boson may be too heavy for the LHC, if it exists :)
good post @lemouth.
Thanks!