Can the Human Brain collapse Quantum Mechanics? - Part 2
As discussed in Part 1, it was argued by John von Neumann that the human brain alone can collapse a wavefunction of a quantum particle, giving it classical properties when observed. In Part 2 I will discuss the opposing views and why I think they are insufficient in describing the collapse postulate.
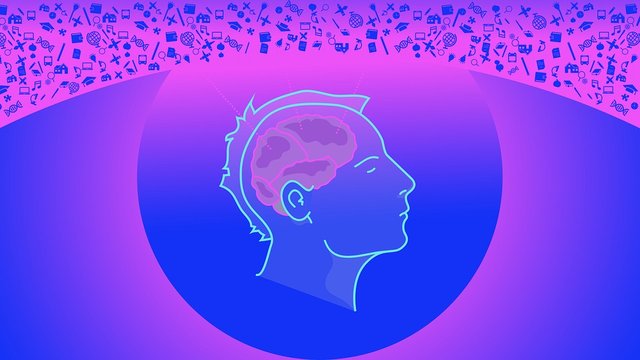
Macroscopic / Microscopic divide
In 1948, Neils Bohr advocated that in the system containing the microscopic particle and the macroscopic apparatus, that the microscopic particle would be governed by quantum mechanics whilst the macroscopic apparatus would be governed by classical mechanics. This means that there is a “distinction” between the two objects as they obey laws of two opposing schools of physics and they cannot be in a quantum mechanical superposition (Bohr, 1948). Essentially, Bohr argued that the apparatus cannot cause the collapse of the particle because a macroscopic, classical apparatus cannot interact a microscopic, quantum particle.
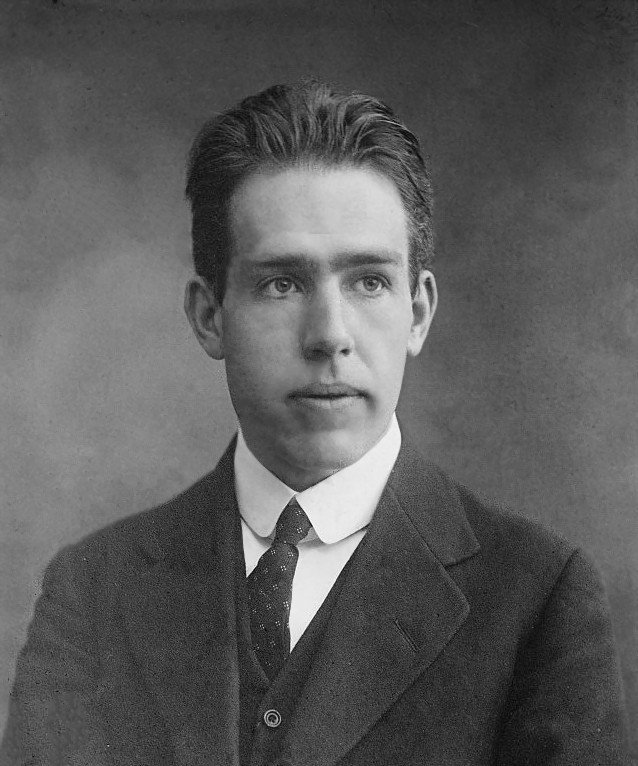
However, this claim is dissolved by employing the infamous Schrödinger’s cat to describe the measurement problem. As shown in figure 1 below, Schrödinger’s cat is in placed in a sealed box containing a radioactive material that has a 50% chance of triggering the gieger counter to break the poison bottle and kill the cat. As the probability of releasing the poison is 50:50, the cat can be thought of as both dead and alive at the same time, so it is in a superposition of the states, dead or alive. An observer has no way of knowing whether the cat is dead or alive whilst the cat is sealed in the box and when one takes a measurement and opens the box to check the cat, the poison is released killing the cat instantly, forcing it into the determinate state of dead (Benich, 2014). As the cat is undeniably a macroscopic object, this thought experiment shows that macroscopic object can behave quantum mechanically as it forms a superposition of states, thus meaning that the apparatus could form a superposition with the particle. Furthermore, Schrödinger’s cat gives the perfect example of the conscious observer that collapses the wavefunction as the cat is in an indeterminate superposition up until the point where the box is opened.
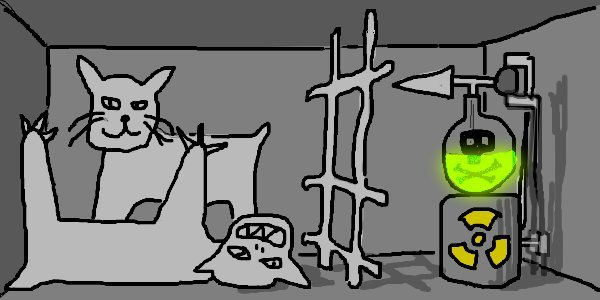
All though Schrödinger’s cat gives a pleasant analogy to describe macroscopic objects behaving quantum mechanically, John Bell points out the problem in describing them in such a way. He ponders; “how exactly is the world to be divided into speak able apparatus ... that we can talk about ... and unspeakable quantum system that we can not talk about?” (Bell, 1986). Essentially, Bell asks where will the line be drawn between classical and quantum or microscopic and macroscopic? At what point do to we start describing objects as quantum or classical? For von Neuman, this line was consciousness.
More problems with measurement
More problems lie in the Schrödinger’s cat analogy of quantum mechanics due to the definition of measurement and whether it impacts on the evolution experiment. As Peter J Lewis points out, “we know from observation that cats are always either dead or alive”. So, at what point during this thought experiment does the seemingly indeterminate cat collapse from being dead and alive to dead or alive? The measurement postulate destroys the quantum mechanical superposition at some point between the radioactive source decaying and the observer revealing the contents of the box, but which exact process defined as the one that breaks the superposition is unclear; providing a failure of Quantum Mechanics (Lewis, 2016).
Furthermore, this leads to the question: what exactly does the term ‘measurement’ mean? Measuring the length of a table with a ruler surely cannot be carried out or explained using the same methods or equipment as, for example, measuring the spin of a quantum particle. However, this is irrelevant because, any of the various pieces of measuring equipment are made of particles, just as the thing it is measuring (Lewis, 2016). This means they must both obey the same quantum mechanical laws, thus forcing them into a superposition. This leads us to conclude that, as there is nothing to separate the measuring equipment and the particle because they are composed of the same material, we seek for something else that does the final measurement to collapse the wavefunction. Although it seems implausible, von Neumann suggested that this ‘something’ was human consciousness. This is hard for us to comprehend that somehow, we can override the laws of quantum mechanics and the next theory I will talk about, GWR, tries to resolve the collapse postulate by eliminating the process of measuring to get rid of these problems.
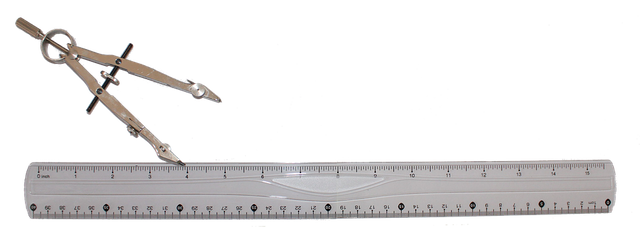
Ghirardi-Rimini-Weber theory
The theory of quantum mechanics describes how a particle can undergo spontaneous collapse with a certain probability. For systems with many particles, if one particle collapses, this collapses the entire system as all particles are entangled in a superposition. Therefore, the likelihood of collapse is high due to the sheer number of particles. Getting rid the observer eliminates the issues with the concept of collapsing a particle with measurement and we do not have to explain what is so special about the measurement that makes the system collapse (Frigg, 2009).
One objection to this theory is due to the Heisenberg uncertainty principle of quantum mechanics. The uncertainty principle tells us that “each post-collapse wavefunction must have some spread in position, so as to avoid infinite spread in momentum (and therefore energy)” (Caulton, 2009). This causes problems for GWR theory as it means that the collapsed particle is not in a definite position so even though the wave function is collapsed, it does not have determinate location as with the measurement solution. This is known as the tails problem.
Another contention to GWR is that it is disagrees with special relativity. All the particles in the system form a superposition with each other; when one particle collapses this causes every other particle in the superposition to also collapse instantaneously and spontaneously. This does not conform to the theory of special relativity as the particles cannot collapse instantaneously over large distances (Caulton, 2009).
The downfalls in GWR theory lead me to conclude that the observer is essential in collapsing the wavefunction and explaining the measurement problem. By eradicating the participator, GWR theory can explain collapse without measurement but it disagrees with too many laws of physics in doing this and so should be disregarded.
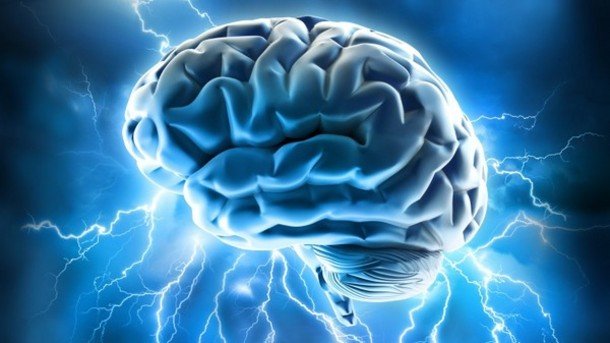
Conclusion
In conclusion, the measurement problem produces a giant loop hole in the theory of quantum mechanics. The collapse of a previously superimposed wavefunction cannot be explained without measurement using human consciousness. It seems somewhat far fetched and astonishing that somehow, humans are superior beings over and above the laws of Physics. The human mind is the only measuring device that doesn’t join the superposition and can collapse the wavefunction as soon as our consciousness registers it.
Please note that images used in this article have been labelled as re-usable on Google images. If any artists or designers have any issues with the content used, don't hesitate to contact me to correct the issue.
References
Bell, J. 1986. Introductory Remarks. [18/01/2018]. Available from: https://www.sciencedirect.com/science/article/pii/0370157386900657
Benich, J. 2014. Bohr and the Measurement Problem: Moving towards Holism in Copenhagen. [18/01/2018]. Available from: https://atrium.lib.uoguelph.ca/xmlui/bitstream/handle/10214/8136/Benich_John_Lawrence_201405_MA%20%282%29.pdf?sequence=1&isAllowed=y
Bohr, N. 1948. On the Notions of Causality and Complementarity. [18/01/2018] Available from: http://www.informationphilosopher.com/solutions/scientists/bohr/Notions_of_Causality_and_Complementarity.pdf
Caulton, A. 2009. Solving the Measurement Problem? Complementarity and dynamical collapse theories. [20/01/2018]. https://www.phil.cam.ac.uk/curr-students/II/II-lecture-notes/ii-p6-jerquantum4.pdf
Frigg, R. 2009. GWR theory. [20/01/2018]. Available from: http://sas-space.sas.ac.uk/1045/1/R_Frigg_GRW2.pdf
Lewis, P. 2016. Quantum Ontology. New York: Oxford University Press.
Being A SteemStem Member
yes please and thank you space nerd here
I have a: #SincechannelDegreeInAstrophysics
thanks @laular for the post/knowledge
:: :: :: :: :: :: :: :: ::
#sincechannel
#astronomy
#science
#physics
#spacenerd4Life
:: :: :: :: :: :: :: :: ::
<3 #kellyshea
Can't wait for the next part :D damned science tells good stories, always wondering what the next theory is going to, what's going to happen next!
Glad you enjoyed! :)